As soon as a star is born, it starts fighting a battle with gravity. A burning star constantly releases enough energy to counteract gravity’s inward pressure. But once its fuel runs out, gravity wins: the star implodes, and most of its mass becomes either a neutron star—an ultradense object about the size of a city—or a black hole. The rest explodes outward, flying into space like bullets.
Astronomers recently captured new images of the aftermath of this violence by training the James Webb Space Telescope (JWST) on the young supernova remnant called Cassiopeia A. The light from its explosion reached Earth about 350 years ago, around the time of Isaac Newton. “This particular object is very important because it’s relatively nearby and it’s young, so what you see is a frozen-in-time picture of how the star blew up,” says Dartmouth College astronomer Robert A. Fesen.
Astronomers have studied this nearby spectacle for decades, but JWST got a closer look than any past observatory. “The Webb images are really amazing,” says Fesen, who led the first team that studied Cassiopeia A with the Hubble Space Telescope. Hubble observes in primarily optical light—the wavelength range human eyes can see whereas JWST captures longer-wavelength infrared light, and it does so with a larger mirror that captures images in higher resolution.
On supporting science journalism
If you’re enjoying this article, consider supporting our award-winning journalism by subscribing. By purchasing a subscription you are helping to ensure the future of impactful stories about the discoveries and ideas shaping our world today.
The recent photographs are helping scientists answer some of their most pressing questions about supernovae, such as which types of stars explode in which ways and how exactly those outbursts unfold. “There is a lot of complicated but beautiful physics in understanding how this explosion takes place,” says Purdue University astronomer Danny Milisavljevic, who led the team behind the JWST images.
Stars start off burning hydrogen into helium inside their fusion furnaces. When the hydrogen is used up, they fuse helium to make carbon, then carbon to make neon, and so on, until they reach iron, which costs more energy to fuse than it releases. At this point the star begins to collapse under gravity, and its matter falls in until most of the protons and electrons inside its atoms have been smushed together to form neutrons. Eventually the neutrons can’t collapse any further—they become a neutron star, where particles experience such extreme pressure that they trigger a repelling shock wave. (Only the most massive stars end their lives in supernovae. The sun, for instance, will fade to become a white dwarf.)
Astronomers still can’t entirely account for the explosive power of a supernova. “It was thought that this rebounding shock that’s produced when the neutron star forms could explode the star,” Milisavljevic says. “But decades of simulations on the world’s fastest computers showed that the rebounding shock isn’t strong enough to overcome the massive layers on top that want to fall in.” For now the core driver of supernova explosions remains a mystery. Researchers suspect the answer involves neutrinos, nearly massless particles that tend to pass through matter unimpeded. Perhaps at the intense temperatures and densities at the core of a star, some of the neutrinos’ energy goes into reviving the shock. But more observations are needed to verify this idea.
Among JWST’s revelations about Cassiopeia A is a layer of gas that escaped its star during the blast. These JWST images show the gas before it interacted with material outside the star and before it was heated by a reflection of the shock wave the star expelled during its eruption. This pristine ejecta from the supernova displays a weblike structure that offers clues about the star before it exploded. “JWST gave us basically a map of the structure of that material,” says Tea Temim, a Princeton University astronomer who collaborated on the JWST images. “This tells us what the distribution of the material was before it was ejected in the supernova. We haven’t been able to see something like this before.”
The investigation also exposed an unexpected feature of Cassiopeia A that scientists have named the “Green Monster.” Astronomers think this layer of gas was expelled by the star before it exploded. “The Green Monster was an exciting surprise,” Temim says. Scientists are interested in what happens when the supernova debris flies into the material in the Green Monster. “This is important,” Temim says, “because when we observe extragalactic supernovae, their light is very much influenced by the surrounding material.”
Deciphering the details of supernovae could even help us understand how Earth and its life came to be. Stars create the elements heavier than hydrogen and helium that life requires. Their end-of-life eruptions spew these elements into space, seeding galaxies with the raw materials to form new stars and planets. “As citizens of the universe, it’s important we understand this fundamental process that makes our place in the universe possible,” Milisavljevic says.
Astronomers will keep studying Cassiopeia A, although their success makes them eager to turn JWST’s eyes toward some of the other roughly 400 identified supernova remnants in our galaxy. Getting a larger sample will help researchers connect differences in how remnants look and evolve to differences among the stars that produced them.
Celestial Firecracker
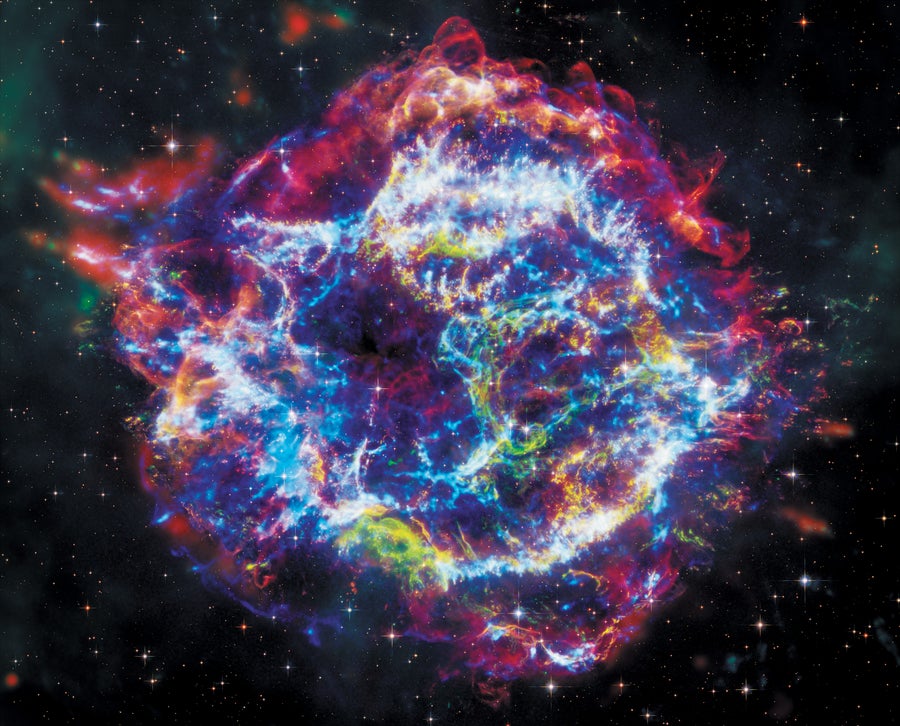
NASA/CXC/SAO (x-ray); NASA/ESA/STScI (optical); NASA/ESA/CSA/STScI/D. Milisavljevic et al., NASA/JPL/Caltech (infrared); NASA/CXC/SAO/J. Schmidt and K. Arcand (image processing)
Cassiopeia A is the aftermath of the closest known young supernova to Earth, a blast that occurred some 350 years ago. Recent data from the James Webb Space Telescope (JWST) combine in this image with earlier observations by the Hubble Space Telescope, the Chandra X-ray Observatory and the Spitzer Space Telescope to reveal a clearer picture of Cassiopeia A than ever before.
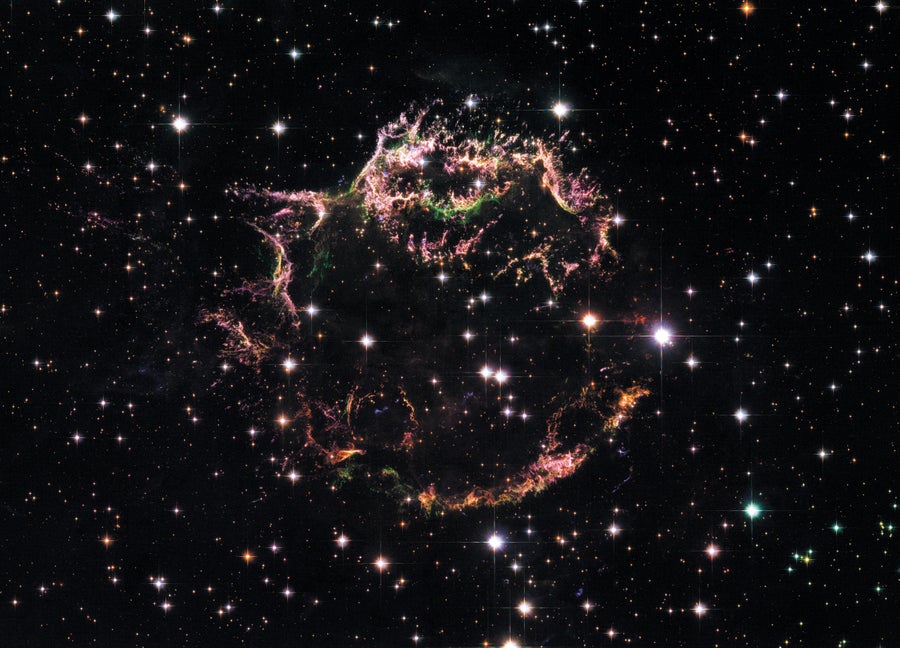
NASA, ESA and Hubble Heritage (STScI/AURA)-ESA/Hubble Collaboration. Acknowledgment: Robert A. Fesen/Dartmouth College and James Long/ESA/Hubble
Before the JWST images, Hubble’s observations of Cassiopeia A were revolutionary. In photographs taken in 2006, Hubble improved on the resolution of ground-based observations by a factor of 10. In the process, it was able to resolve clumps of material ejected during the supernova that were traveling shockingly fast, between 8,000 and 10,000 kilometers per second. “The explosion is ridiculously violent,” Fesen says. “The outer layers of the star appear to fragment into clumps of gas, almost like the star shattered into thousands and thousands of pieces.” Scientists hadn’t realized that the blast would produce such clumps, Fesen says. “Nature had to show us that stars actually do that.”
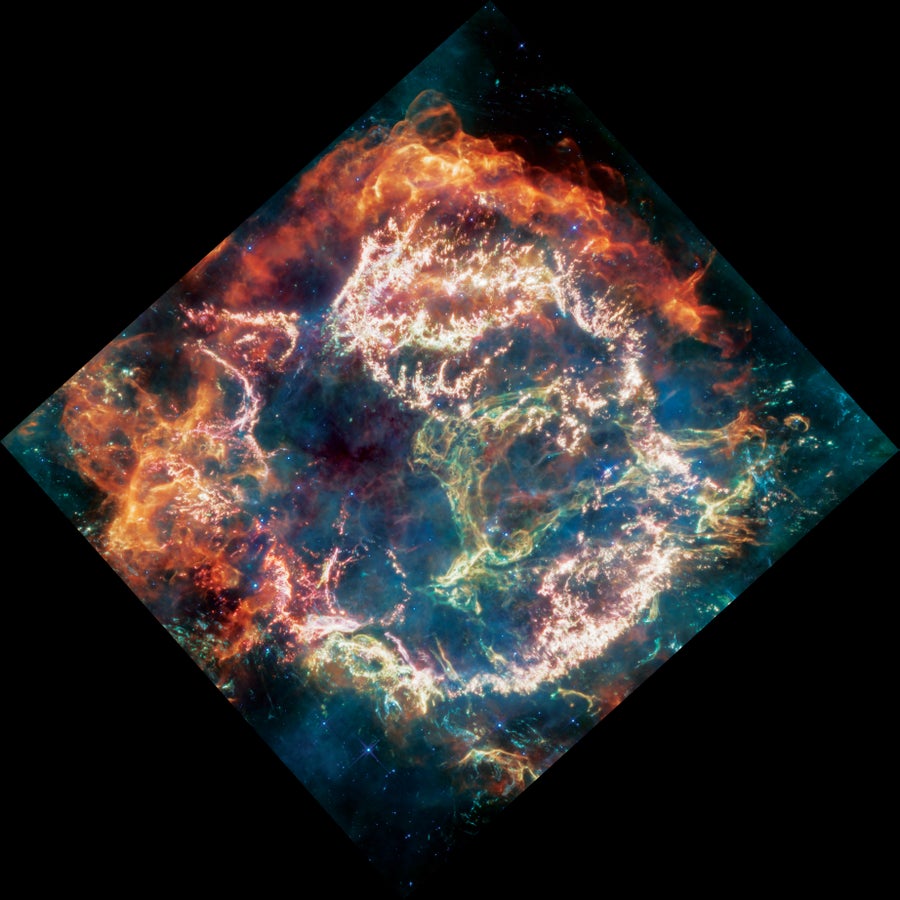
NASA, ESA, CSA, Danny Milisavljevic/Purdue University, Tea Temim/Princeton University, Ilse De Looze/University of Ghent; Joseph DePasquale/STScI (image processing)
JWST is the most powerful telescope of all time, and its portrait of Cassiopeia A shows never-before-seen details. The observatory’s Mid-Infrared Instrument (MIRI) captures various bands of infrared light, which have each been converted into respective visible-light colors in this picture. Orange and red flows on the top and left of the image show spots where material from the exploding star is smashing into gas and dust in the surrounding area. Inside this shell are bright pink strands released during the explosion. The dark red web toward the center left represents pristine structure from the blast that could hold clues about the star before it blew up.
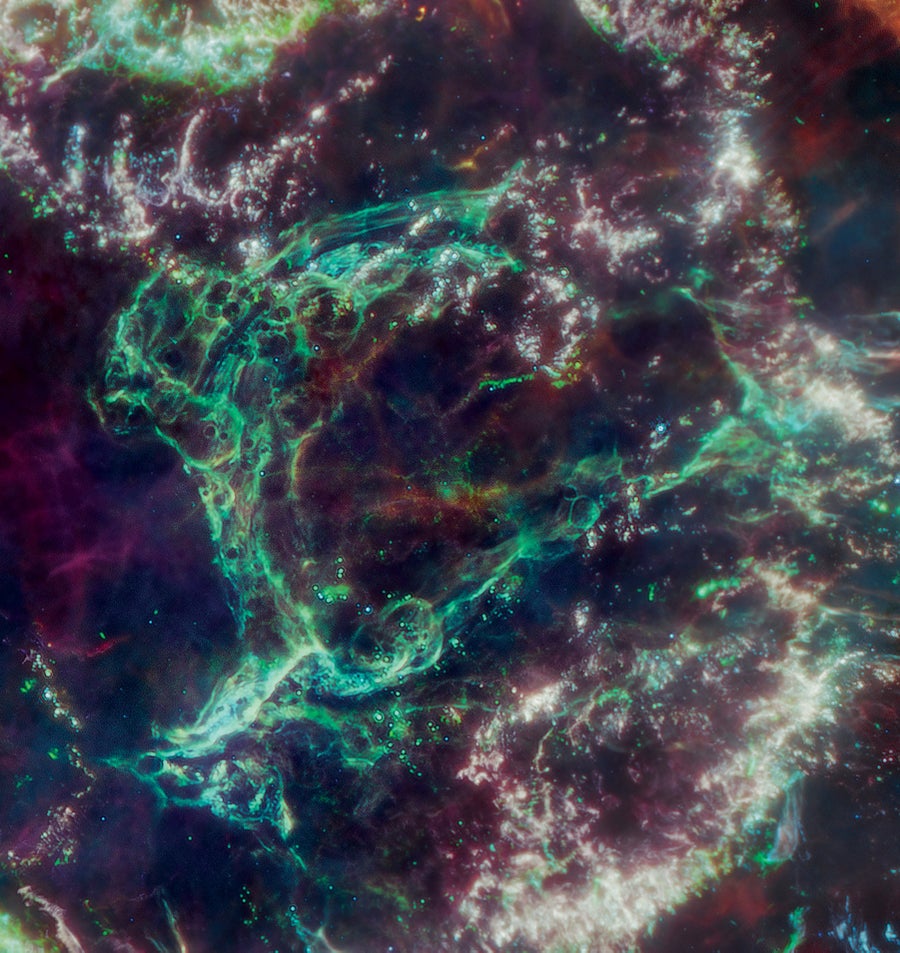
NASA/ESA JWST, Danny Milisavljevic/Purdue University, Tea Temim/Princeton University, Ilse De Looze/University of Ghent and HST, R. Fesen/Dartmouth College; J. Schmidt (image processing)
Zooming in on the JWST image reveals a surprise—a green bubble scientists are calling the “Green Monster” after a green wall at Fenway Park in Boston. This blob is made of gas layers the star cast off before it burst apart. “It looks weird and has this bizarre distribution of rings and filaments,” Milisavljevic says. “Encoded in this puzzle is information about how the star was releasing mass before the explosion.”
Holes apparent in the Green Monster seem to provide evidence of the clumps of ejecta Fesen and his team observed with Hubble. “The images from JWST show little holes, almost like bullet holes, that are almost perfectly round,” he says. Scientists think the fast-moving clumps of supernova material are punching through the surrounding sheet of gas like shrapnel to create the holes. The size of the holes betrays the clumps’ gigantic size—roughly 500 astronomical units (the distance between Earth and the sun). “As these clumps have been sailing through space, they’ve expanded to become bigger than the solar system,” Fesen says.
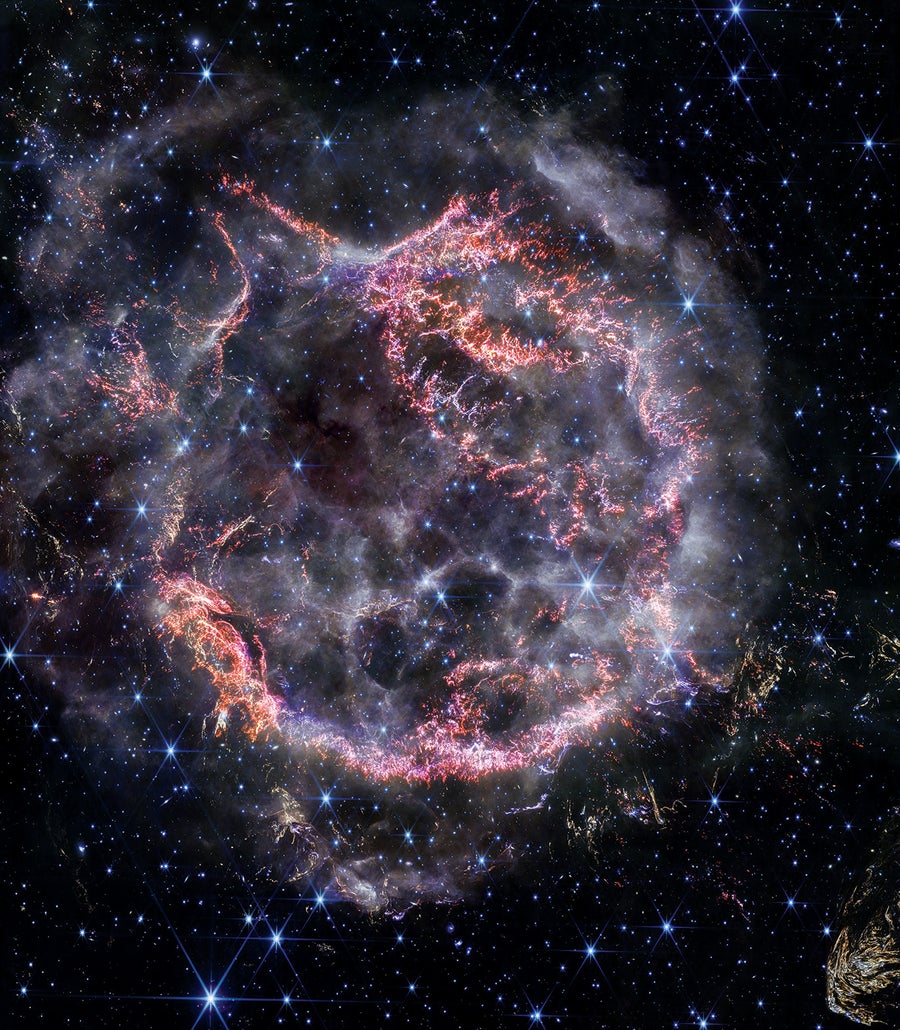
NASA, ESA, CSA, STScI, Danny Milisavljevic (Purdue University), Ilse De Looze (UGent), Tea Temim (Princeton University)
Another JWST instrument, the Near-Infrared Camera (NIRCam), showcases Cassiopeia A in shorter-wavelength light than MIRI. “The benefit of NIRCam is resolution,” Milisavljevic says. “When you zoom in like this, it’s astounding. I’m going to spend the rest of my career trying to understand the supernova at these scales.” He hopes to use these data to understand how the shock wave of the explosion has shaped the gas it encountered, as well as how dense the supernova material can get, to garner clues about how the cataclysm unfolded.